
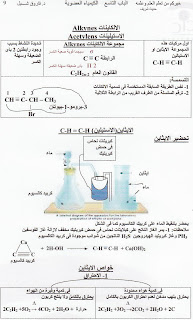
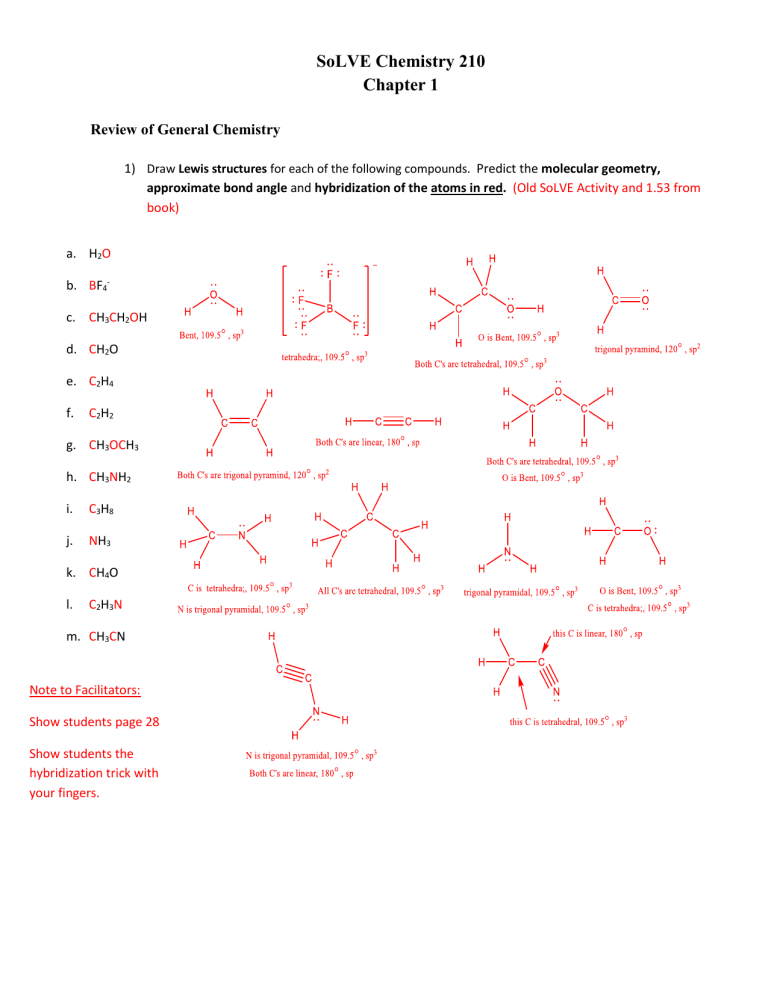
The difference, of course, is that the larger alcohols have larger nonpolar, hydrophobic regions in addition to their hydrophilic hydroxyl group. What is happening here? Clearly, the same favorable water-alcohol hydrogen bonds are still possible with these larger alcohols. The longer-chain alcohols - pentanol, hexanol, heptanol, and octanol - are increasingly non-soluble. When you try butanol, however, you begin to notice that, as you add more and more to the water, it starts to form its own layer on top of the water. This is because the water is able to form hydrogen bonds with the hydroxyl group in these molecules, and the combined energy of formation of these water-alcohol hydrogen bonds is more than enough to make up for the energy that is lost when the alcohol-alcohol hydrogen bonds are broken up. You find that the smaller alcohols - methanol, ethanol, and propanol - dissolve easily in water.
#TEXTLAB ORGANIC CHEMISTRY CHAPTER 9 SERIES#
Next, you try a series of increasingly large alcohol compounds, starting with methanol (1 carbon) and ending with octanol (8 carbons). Water is a terrible solvent for nonpolar hydrocarbon molecules: they are very hydrophobic (water-hating). Thus, the energetic cost of breaking up the biphenyl-to-biphenyl interactions in the solid is high, and very little is gained in terms of new biphenyl-water interactions. It is able to bond to itself very well through nonpolar van der Waals interactions, but it is not able to form significant attractive interactions with the very polar solvent molecules. Why is this? Because it is a very non-polar molecule, with only carbon-carbon and carbon-hydrogen bonds. Now, we’ll try a compound called biphenyl, which, like sodium chloride, is a colorless crystalline substance (the two compounds are readily distinguishable by sight, however – the crystals look quite different).īiphenyl does not dissolve at all in water.
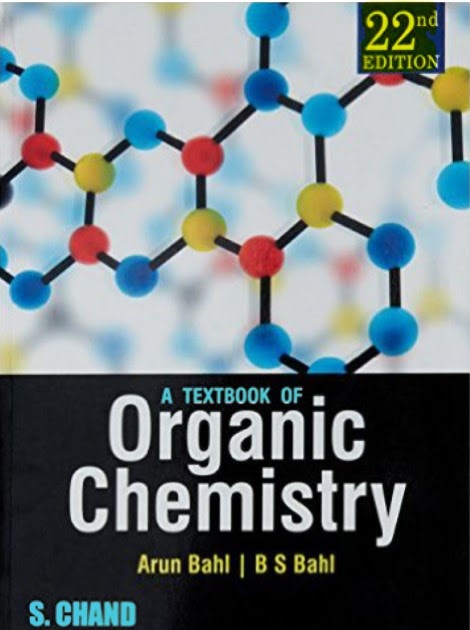
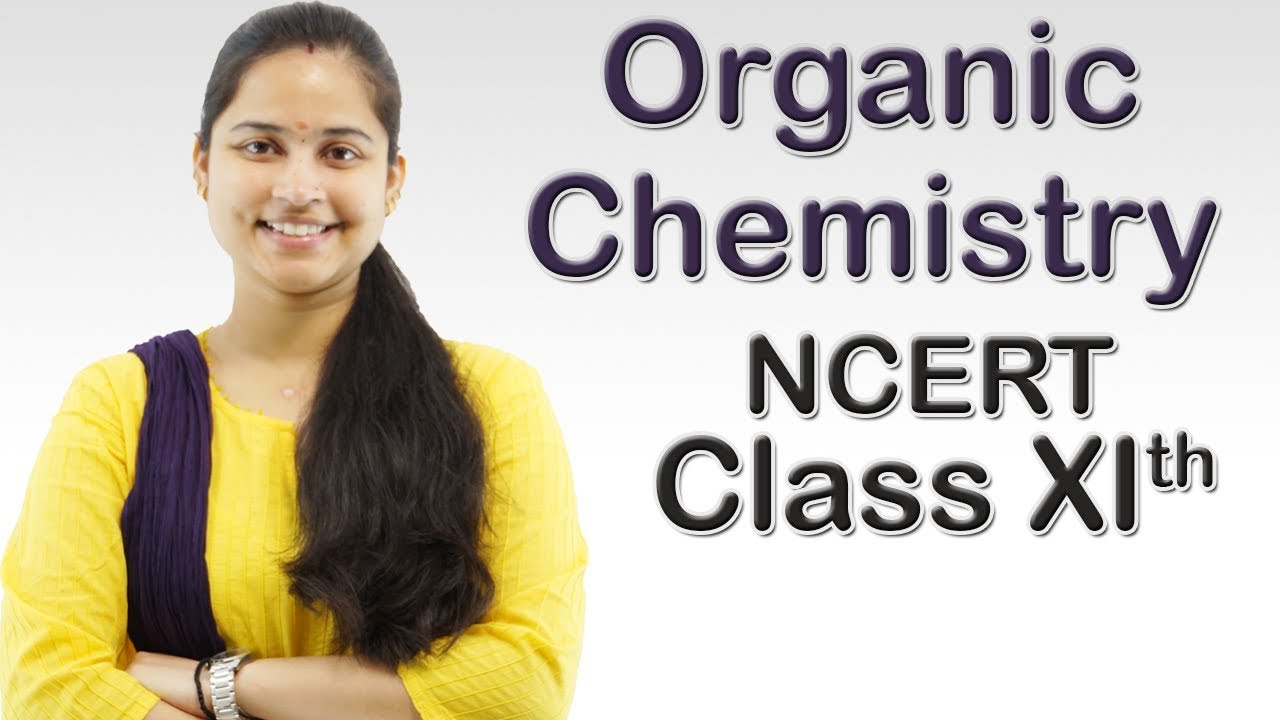
Charged species as a rule dissolve readily in water: in other words, they are very hydrophilic (water-loving). The end result, then, is that in place of sodium chloride crystals, we have individual sodium cations and chloride anions surrounded by water molecules – the salt is now in solution. Why? Because water, as a very polar molecule, is able to form many ion-dipole interactions with both the sodium cation and the chloride anion, the energy from which is more than enough to make up for energy required to break up the ion-ion interactions in the salt crystal and some water-water hydrogen bonds. As you would almost certainly predict, especially if you’ve ever inadvertently taken a mouthful of water while swimming in the ocean, this ionic compound dissolves readily in water. The first substance is table salt, or sodium chloride. Imagine that you have a flask filled with water, and a selection of substances that you will test to see how well they dissolve in the water. Let’s revisit this old rule, and put our knowledge of covalent and noncovalent bonding to work. You probably remember the rule you learned in general chemistry regarding solubility: ‘like dissolves like’ (and even before you took any chemistry at all, you probably observed at some point in your life that oil does not mix with water). It is critical for any organic chemist to understand the factors which are involved in the solubility of different molecules in different solvents. In organic reactions that occur in the cytosolic region of a cell, the solvent is of course water. In recent years, much effort has been made to adapt reaction conditions to allow for the use of ‘greener’ (in other words, more environmentally friendly) solvents such as water or ethanol, which are polar and capable of hydrogen bonding. In the organic laboratory, reactions are often run in nonpolar or slightly polar solvents such as toluene (methylbenzene), hexane, dichloromethane, or diethylether. Virtually all of the organic chemistry that you will see in this course takes place in the solution phase.
